Electrocatalysis: A Key Technology for Energy Storage and Conversion
As the world transitions towards renewable energy, electrocatalysis has become a crucial technology for energy storage and conversion. Whether in fuel cells, metal-air batteries, water electrolysis for hydrogen production, or CO₂ reduction (CO₂RR), electrocatalysis plays a fundamental role in enhancing energy efficiency and reducing costs. However, compared to traditional catalysis methods, electrocatalysis is significantly more complex, requiring advanced materials and precise control over reaction conditions.
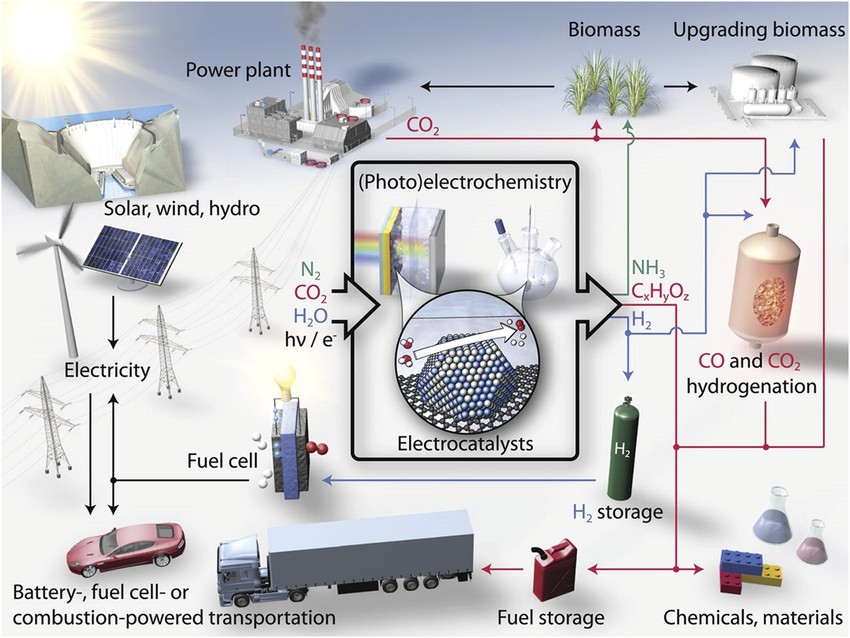
(Source: Schematic of a sustainable energy landscape based on electrocatalysis)
The Role of Electrocatalysis in Energy Storage and Conversion
Electrocatalysis accelerates chemical reactions using electric energy, facilitating the efficient conversion and storage of renewable energy. Some of the most critical energy-related electrocatalytic reactions include:
1.ORR in Fuel Cells & Metal-Air Batteries
- ORR(Oxygen Reduction Reaction) occurs at the cathode of fuel cells and metal-air batteries, determining their efficiency and power output.
- Fuel cells (e.g., PEMFC, SOFC) power electric vehicles and portable energy systems.
- Zn-air and Li-air batteries rely on ORR for high energy density storage solutions.
2.OER in Water Electrolysis & Rechargeable Batteries
- OER(Oxygen Evolution Reaction) is the critical reaction in water splitting, where electricity is used to generate green hydrogen.
It is also essential for charging metal-air batteries, impacting their rechargeability and lifespan.
3.HER in Water Splitting
HER(Hydrogen Evolution Reaction) is the cathodic reaction in electrolysis, where hydrogen gas is produced from water, forming the foundation of a hydrogen-based economy.
4.CO₂RR for Carbon Neutrality
- CO₂RR(CO₂ Reduction Reaction) electrochemically converts CO₂ into valuable chemicals such as methanol, ethanol, and carbon monoxide, contributing to carbon capture and utilization (CCU).
These electrocatalytic processes are vital for improving energy efficiency, reducing carbon emissions, and enabling large-scale renewable energy adoption.
Electrocatalysis vs. Other Catalysis Methods
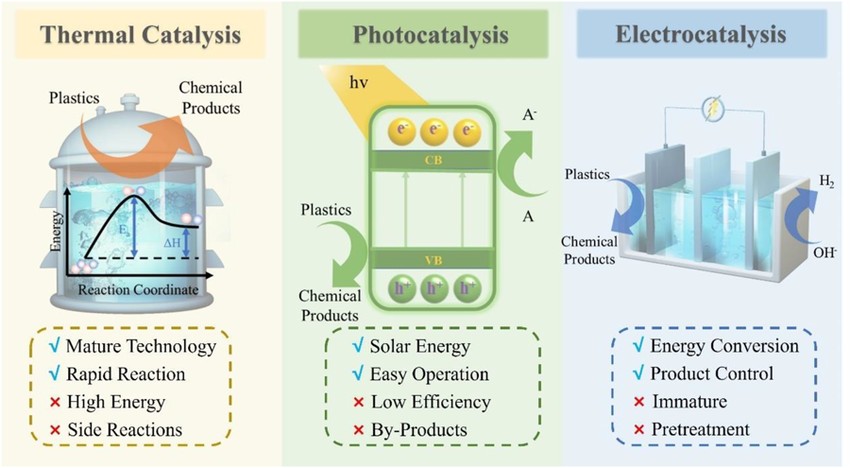
(Source: Advantages and limitations of thermal catalysis, photocatalysis, and electrocatalysis)
Catalysis is a key concept in modern industry, energy, and environmental science. However, different catalytic methods rely on distinct energy sources:
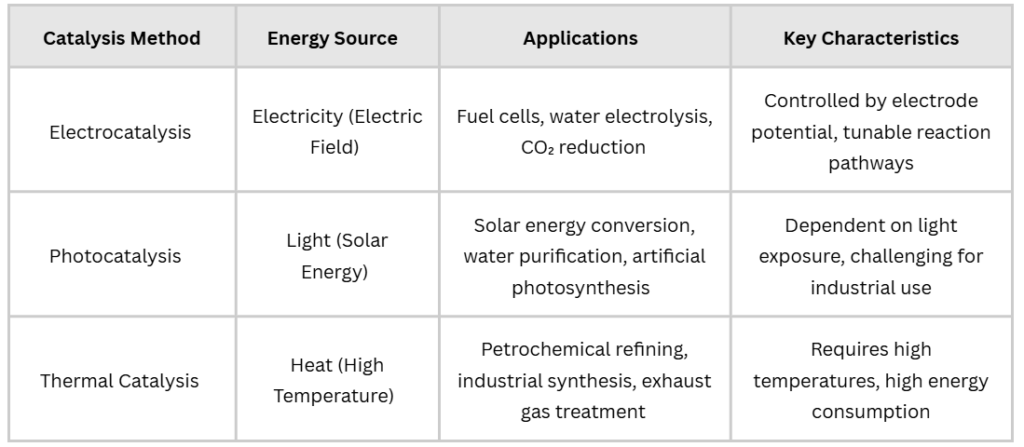
Similarities
- All three methods use catalysts to lower reaction barriers and accelerate reaction rates.
- They require careful tuning of catalyst properties such as activity, stability, and selectivity.
- Advances in materials science and surface chemistry have improved catalyst performance across all fields.
Differences
1.Energy Source & Efficiency
- Thermal catalysis relies on high temperatures (e.g., Haber-Bosch process for ammonia synthesis).
- Photocatalysis requires specific light conditions (e.g., TiO₂-based solar-driven water splitting).
- Electrocatalysis operates under electrochemical control, allowing precise reaction tuning.
2.Reaction Environment
- Thermal catalysis mainly occurs in gas or liquid phase at high temperatures.
- Photocatalysis is light-dependent, requiring materials with appropriate band gaps.
- Electrocatalysis occurs at the electrode-electrolyte interface, involving electron and ion transport.
3.Controllability & Versatility
- Electrocatalysis offers higher control over reaction pathways by adjusting potential, current, and pH, making it highly versatile for energy conversion applications.
What Do We Need to Study Electrocatalysis?
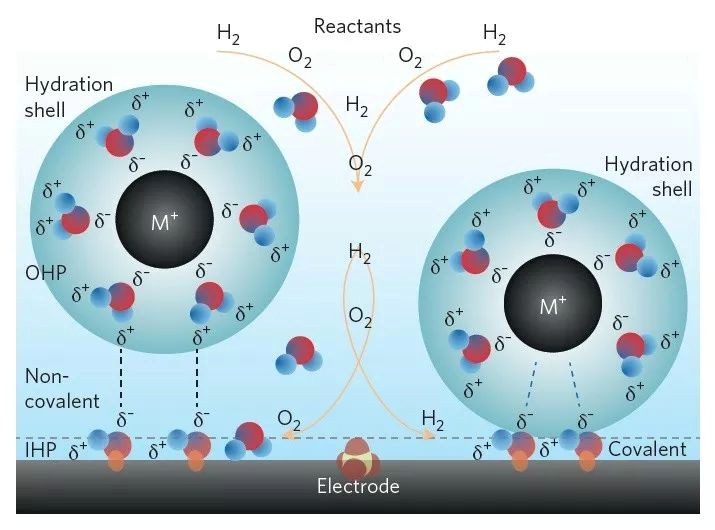
(Source: Energy and fuels from electrochemical interfaces)
To understand electrocatalysis, we must examine the electrode/solution interface and the reaction pathway it follows. The process begins with:
Step 1: Reactant Diffusion – The process begins with reactants diffusing to the electrode surface, a step controlled by mass transport in the electrolyte.
Step 2: Electron Transfer or Adsorption – Once at the surface, reactants may either undergo direct electron transfer (outer-sphere reaction) or adsorb onto the electrode surface (inner-sphere reaction), where solvent restructuring can influence reaction kinetics.
Step 3: Core Electrocatalytic Reactions – This is the key step in electrocatalysis, involving a series of elementary processes such as adsorption, dissociation, electron transfer, and surface diffusion. These reactions determine the overall efficiency and selectivity of the system.
Step 4: Product Desorption and Transport – Finally, the reaction products detach from the surface and diffuse into the bulk solution, completing the catalytic cycle.
To better study electrocatalysis, what do we need to do?
1. Understanding the Electrode/Solution Interface
The interface between the electrode and solution plays a crucial role in electrocatalysis, influencing reaction kinetics and selectivity. Several key factors require investigation:
- The electric double layer—how it affects potential distribution, reactant concentration, and surface chemistry.
- Solvent restructuring—its impact on reaction intermediates and overall catalytic efficiency.
- Surface adsorption effects—how organic molecules on nanoparticles influence catalytic activity.
2. Developing Advanced Atomic-Scale Characterization Tools
To understand how catalysts behave during reactions and identify the true active sites, we need high-resolution analytical techniques:
- Atomic-scale imaging: STM (Scanning Tunneling Microscopy), AFM (Atomic Force Microscopy), XPS (X-ray Photoelectron Spectroscopy).
- In situ/operando analysis: Synchrotron-based XRD, in situ Raman, XAS (X-ray Absorption Spectroscopy).
3. Investigating the “Surface Active Region” Concept
Traditionally, catalyst studies have focused on active sites, but recent research suggests that a broader surface active region—including solvents, adsorbed species, and transition states—plays a decisive role.
- Key questions:
- How can we experimentally verify these effects?
- How can we quantify the cooperative effects between surface species using theoretical models?
4. Quantifying and Optimizing Catalyst Performance
To improve electrocatalysts, we need methods to quantify their active regions and optimize their efficiency.
- Core challenges:
- How can we comprehensively evaluate both the number and activity of surface active regions?
- How can we combine experimental techniques with theoretical calculations to quantify reaction mechanisms?
- Potential solutions:
- Machine learning + DFT (Density Functional Theory) + High-throughput catalyst screening.
- Combining Electrochemical Impedance Spectroscopy (EIS) with in situ spectroscopic techniques to analyze reaction dynamics at active sites.
Electrocatalysis plays a critical role in advancing energy storage and conversion technologies, but truly understanding its mechanisms requires a comprehensive approach. At ANR, We strive to provide researchers with the right tools, materials, and support to explore reaction mechanisms, optimize catalyst performance, and advance energy solutions. Whether it’s electrochemical testing, high-quality materials, we are here to help researchers drive innovation and contribute to a cleaner, more sustainable energy future.
Other Blog Posts You Might Like
Key Characterization Techniques in Battery Research
In battery materials research, structure, composition, and performance are closely interrelated. To accurately analyze and…
Read moreCompany insight: Strengthening capability Through Strategic Partnerships
At ANR, our commitment to innovation and excellence is driven by the quality of our…
Read moreCase Study: Relocation Solution for Electrochemical Equipment
Project Overview A research institution faced the daunting task of relocating two vital laboratory furnaces.…
Read more